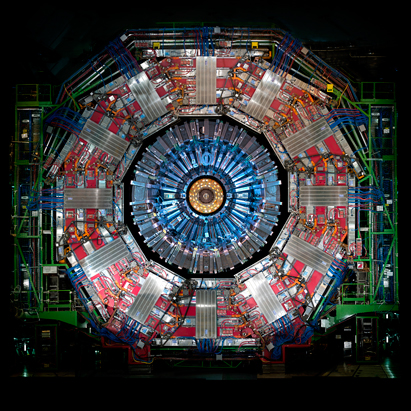
Experimental Particle Physics at The University of Alabama consists of seven faculty working on:
- Collider physics research with the CERN CMS experiment
- Direct dark matter search with the LZ experiment, and
- Multiple groups focused on neutrino projects with KamLAND, MiniBooNe, EXO-200, nEXO, Double Chooz, and ν – SNS. These groups are currently members of several different international collaborations dedicated to studying neutrinos.
- Other physics being looked into by these research groups include: supernovae, neutron decay, neutrino magnetic moments, geo-neutrinos, negative muon capture, magnetic monopoles, and more.
Collider Physics Group
Research activities in the Collider Physics group at The University of Alabama are focused on the Compact Muon Solenoid (CMS) experiment at the Large Hadron Collider (LHC) in CERN, the European particle physics laboratory in Geneva, Switzerland. The UA Collider Physics group is led by Dr. Paolo Rumerio, Dr. Sergei Gleyzer, and Dr. Emanuele Usai.
The CMS Collaboration is an international collaboration of over 2000 scientists who operate the Compact Muon Solenoid detector at the Large Hadron Collider in CERN. The LHC is colliding protons at the highest energies ever achieved in the laboratory. These collisions have already discovered the long-elusive Higgs boson, and it is hoped that they may also reveal even more exotic new physics, such as supersymmetry or extra dimensions of space. For more information on the Alabama group’s activities on CMS, visit the UA Collider Physics group webpage.
Neutrino Projects
KamLAND is a 1000 ton liquid scintillation detector in the Kamioka mine in Japan. KamLAND has been observing anti-neutrinos from the nuclear reactors in the Asian region and played an important role in understanding the phenomenon of neutrino flavor oscillations. By knowing the power of each of the nuclear reactors in the region, the total flux of anti-neutrinos can be deduced. From this total flux KamLAND looks for a deficit in the number of electron anti-neutrinos that it sees. The KamLAND collaboration also developed techniques to purify the liquid scintillator from radio-isotopes to make it possible to see the Be-7 solar neutrino. The purification techniques were developed extensively at Alabama, CalTech, and Tohoku University. Ask Dr. Piepke to learn more about the KamLAND experiment.
MiniBooNE is a 800 ton liquid scintillation detector at Fermilab, situated down-line from the 8 GeV proton accelerator. There it converts protons into pions by colliding the protons into a beryllium target. The pions are unstable and decay into muon and muon neutrino pairs. The muons interact well with matter and can easily be stopped by a steel absorber. The neutrinos, however, have a very small cross section which allows nearly all of them to pass unhindered through the steel and into the detector. Here MiniBooNE looks to observe the appearance of electron type neutrinos which oscillated from the muon neutrino. MiniBooNE has a unique capability of observing both muon neutrinos and muon anti-neutrinos by focusing the positive and negative pions produced in the collision with the target by a large magnetic field. By changing the magnetic field they are able to focus the negative pions, which decay into negative muons and muon anti-neutrinos, toward the detector. MiniBooNE is looking to uncover the existence of a hypothetical fourth non-interacting sterile neutrino, existence of which was suggested by the LSND measurement.
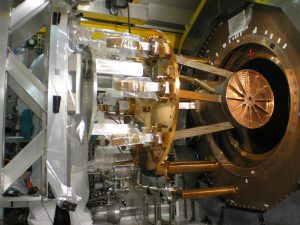
EXO-200 uses enriched liquid Xenon to search for the neutrinoless double beta decay. Existence of this decay will prove that neutrinos are their own anti-particles, which would make them the only known Majorana fermion. EXO-200 has discovered the two-neutrino mode of the double beta decay in Xe-136 and established stringent limits on the neutrinoless mode. Its last search for the neutrinoless double beta decay was published in Nature. We played important role in preparation of the experiment and made leading contributions to the data analysis. While EXO-200 will continue taking data for several more years, we are already working on the next generation experiment, nEXO. nEXO will contain 5 tonne of enriched xenon. It will be located 2 miles underground, in the SNOLAB facility in Canada. Ask Dr. Piepke and Dr. Ostrovskiy about the double beta decay program at the UA.
Double Chooz is a neutrino oscillation experiment designed to measure the last unknown neutrino mixing angle ϑ13. It used the site that is home to the former CHOOZ experiment in France. The measurement process involves the correlation of events from a near and far liquid scintillation detectors. This allows one to study neutrino oscillations with a much smaller systematic error, as compared to a single detector. Double Chooz published its first result in 2012, suggesting a non-zero value of the ϑ13. The UA group played the key role in this result, from the calibration, to determination of major systematic uncertainties, to the final statistical analysis. The paper received more than 1000 citations to date. Ask Dr. Stancu, Dr. Busenitz, and Dr. Ostrovskiy about the neutrino oscillation program at the UA.
ν – SNS will be situated at Oak Ridge National Laboratories at the Spallation Neutron Source (SNS) which is currently under construction. Once construction on the SNS is complete a neutrino detector will be placed here designed specifically to measure the neutrino-nucleus cross sections of importance to current neutrino physics and cosmology. The project will be complete in approximately three years after SNS is is completed and it is proposed that the first neutrino-nucleus cross section can be measured to within an accuracy of 10% within the first year of operation. Ask Dr. Stancu for more details.
Other Research Groups
The group headed by Dr. Jerry Busenitz currently focuses on the LZ experiment. It also played a significant role in analyzing the calibration data for the KamLAND detector and the design and certification of its calibration sources. The group consists of a Post-Doctoral Researcher and two Graduate Research Assistants. Dr. Busenitz was also involved in the development of Double Chooz experiment. This work consisted of performing low-background counting of material samples and Monte Carlo simulation of detector designs.
The group headed by Dr. Juijen (Ryan) Wang is working on developing detector techniques for direct dark matter detection. He is currently involved in LZ direct dark matter detection using a dual-phase liquid xenon Time Projection Chamber (TPC) and a liquid scintillator-based outer detector. He is also involved in the new generation of the veto detector for future dark matter or neutrino experiments.
The group headed by Dr. Ion Stancu is working on the LZ and MiniBooNE experiments. It consists of one Post-Doctoral Researcher who is working on site at FermiLab and two Graduate Research Assistants. Dr. Stancu is also working on the Double Chooz and ν – SNS experiments.
The group headed by Dr. Andreas Piepke is currently working on two major projects, KamLAND and EXO. The work for KamLAND consists of developing techniques to remove 85Kr, 210Pb, 210Po and 222Rn from the liquid scintillator and balloon surface. The main focus of work on KamLAND is to better understand the current low-background signal in KamLAND so a 7Be signal can be seen after a purification of the 1000 tons of liquid scintillator.
Dr. Piepke’s group also has the capabilities of performing ultra sensitive measurements of radio-isotopes by using neutron activation analysis. This is a method in which neutrons are used to excite the material’s isotopes, which then subsequently decay, and the parent nuclei are detected in a low background germanium detector. By knowing the flux of neutrons from the reactor a measurement of radio-isotopic abundance is calculated. This capability is used to screen materials for use in KamLAND and EXO. Other detection capabilities consist of a second germanium detector for counting high activity samples, an alpha detector and a 212Bi – 212Po coincidence setup.
The main work being performed for EXO is in screening materials for the development of the detector and in running Monte Carl simulations. The Monte Carlo is of specific detector designs and consists of determining what background would be seen given the measured material isotopic abundance and external (muon, rock, etc) backgrounds. The group consists of one Post-Doctoral Researcher and two Graduate Research Assistants.
Dr. Ostrovskiy‘s two primary research foci are search for magnetic monopoles and R&D for the next-generation liquified noble gas experiments searching for neutrinoless double beta decay and dark matter. For the up-to-date information about the group’s research and opportunities to participate, please visit the group’s website.
In addition to the faculty mentioned above, the following faculty contribute to the particle physics group:
Dr. Dawn Williams is developing techniques to identify tau neutrinos detected by IceCube, which is the largest neutrino detector on Earth and is located at the South Pole.
Dr. Marcos Santander is interested in high-energy neutrino astrophysics and multi-messenger searches for neutrino sources using gamma-ray and X-ray telescopes. He is involved with IceCube and the Very Energetic Radiation Imaging Telescope Array System (VERITAS), a ground-based gamma-ray instrument operating at the Fred Lawrence Whipple Observatory (FLWO) in southern Arizona, USA