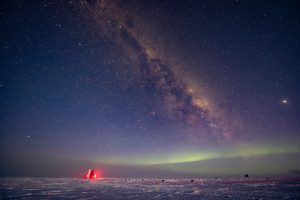
From the UA News Center | Two researchers from The University of Alabama are part of an international project that detected a cosmic interaction never observed before, but predicted 60 years ago.
Drs. Dawn Williams and Marcos Santander, both in the UA Department of Physics and Astronomy, are co-authors on a paper published in Nature that details how a massive telescope buried in an Antarctic glacier detected the moment a high-energy particle called an electron antineutrino, hurtling to Earth from outer space at close to the speed of light, smashed into an electron and produced a particle that quickly decayed into a shower of secondary particles.
Through the IceCube Neutrino Observatory, scientists had seen for the first time a Glashow resonance event, a phenomenon predicted by Nobel laureate physicist Sheldon Glashow in 1960. With this detection, scientists provided another confirmation of the standard model of particle physics. It also further demonstrated the ability of IceCube, which detects nearly massless particles called neutrinos using thousands of sensors embedded in the Antarctic ice, to do fundamental physics.
“This observation is not just a validation of a prediction made over 60 years ago, but it can also tell us something about the mechanisms at work in the cosmic objects producing these neutrinos,” said Santander, an assistant professor.
The event happened in December 2016, but it took years to study and analyze. Williams was analysis coordinator for IceCube from 2017-2019, responsible for organization and management of all data analysis efforts in the project, including the Glashow resonance event. Santander serves on the project’s realtime oversight committee and the publications committee that oversees and coordinates submissions of findings from the project.
The IceCube Collaboration is an international project with over 400 scientists, engineers and staff from 53 institutions in 12 countries, working on the observatory, an array of 5,160 basketball-sized optical sensors deeply encased within a cubic kilometer of clear Antarctic ice. The observatory was set up to detect neutrinos, abundant subatomic particles famous for passing through anything and everything, rarely interacting with matter. About 100 trillion neutrinos pass through our bodies every second.
Since IceCube started full operation in May 2011, the observatory has detected hundreds of high-energy astrophysical neutrinos and has produced a number of significant results in particle astrophysics, including the discovery of an astrophysical neutrino flux in 2013 and the first identification of a source of astrophysical neutrinos in 2018. But the Glashow resonance event is especially noteworthy because of its remarkably high energy; it is one of the three highest energy events ever detected by IceCube.
“The significance of this finding is that this is the first analysis which can specifically identify an antineutrino, the antimatter counterpart of the neutrino, and this gives us insight into the environment in which these incredibly high energy particles are produced,” said Williams, a professor.
Glashow first proposed this resonance in 1960 in a paper in which he predicted an antineutrino, a neutrino’s antimatter twin, could interact with an electron to produce an as-yet undiscovered particle — if the antineutrino had just the right energy — through a process known as resonance.
When the proposed particle, the W-boson, was discovered in 1983, it turned out to be heavier than expected. The Glashow resonance would require a neutrino with an energy almost 1,000 times more energetic than any human-made particle accelerator is capable of producing.
But what about a natural accelerator — in space? The enormous energies of supermassive black holes at the centers of galaxies and other extreme cosmic events can generate particles with energies impossible to create on Earth. Such a phenomenon was likely responsible for the antineutrino that reached IceCube in 2016.
“When Glashow was a postdoc at Niels Bohr, he could never have imagined that his unconventional proposal for producing the W- boson would be realized by an antineutrino from a faraway galaxy crashing into Antarctic ice,” says Dr. Francis Halzen, professor of physics at the University of Wisconsin–Madison, the headquarters of IceCube maintenance and operations, and principal investigator of IceCube.
The result also opens up a new chapter of neutrino astronomy because it starts to disentangle neutrinos from antineutrinos. To confirm the detection and make a decisive measurement of the neutrino-to-antineutrino ratio, the IceCube Collaboration wants to see more Glashow resonances. A proposed expansion of the IceCube detector, IceCube-Gen2, would enable the scientists to make such measurements in a statistically significant way. The collaboration recently announced an upgrade of the detector that will be implemented over the next few years, the first step toward IceCube-Gen2.
Williams plays a key role in calibration and characterization efforts for the upgrade.